|
Essentials in anatomy and physiology of the adenohypophysis:
| | | 
Three efferent systems emerge from the
brain and spinal cord. Two are neural-the somatic and visceral efferent
systems. The third is humoral -the neuroendocrine system. The somatic
efferent system employs striated muscle cells to move the organism or its
component parts in response to environmental stimuli. The visceral efferent
system employs smooth muscle cells and exocrine gland cells to regulate
vascular function and to assimilate nutrients. The neuroendocrine system
employs the pituitary gland to regulate the function of selected visceral
organs (kidney, uterus, and breast), to regulate growth, and to trophically
support and regulate the function of specific target organs of internal
secretion the thyroid gland, adrenal gland, and gonads. Through the
pituitary gland the brain affects the growth and development of the
organism, maintains its internal milieu, regulates its metabolism, and
assures its reproduction.
The Adenohypophysis
The pituitary
gland is also called the hypophysis cerebri (from the Greek hypo-, below,
and phuein, to grow). It is composed of glandular and neural tissue and
hence is subdivided into an adenohypophysis and a neurohypophysis. The
glandular cells that make up the adenohypophysis may be viewed as the
effector cells of the neuroendocrine system. They perform this role by
synthesizing protein hormones and secreting them into nearby capillaries.
The adenohypophysis is not generally believed to be a neural diverticulum
but is thought to arise instead from the primitive foregut-the stomodeum. A
diverticulum of the stomodeum is believed to migrate cranially, pinch off
from the foregut, and become applied to a neural diverticulum emerging from
the diencephalon- the neurohypophysis. With the establishment of vascular
connections between the neurohypophysis and the displaced foregut tissue,
differentiation of the stomodeal remnant into the adenohypophysis occurs.
However, this classic scheme has been challenged with the recognition that
some adenohypophyseal cells contain S-100 protein and others are capable of
amine precursor uptake and decarboxylation and thus can be considered as
part of the APUD system. Cells in the APUD system are believed to be of
neural origin (frequently originating from the neural crest). Takor and
Pearse have suggested that the "ventral neural ridge" gives rise to the
adenohypophysis and thus that the adenohypophysis is of neuroectodermal, not
stomodeal, origin. The finding that some hypothalamic neurons are capable of
synthesizing adrenocorticotropic hormone and melanocyte-stimulating hormone
(hormones that are also synthesized in adenohypophyseal cells) strengthens
the argument that at least some adenohypophyseal cells migrate from the
brain to the adenohypophysis. The observation that adenohypophyseal cells
look epithelial (glandular) does not vitiate the argument, as adrenal
medullary cells (which are neural crest derivatives) are also glandular in
appearance. However, little supportive evidence for a neural origin of the
adenohypophysis has emerged subsequently. An ectodermal origin, similar to
that of the salivary glands, seems likely for most elements in the
adenohypophysis.
The
adenohypophysis is made up of connective tissue, fenestrated capillaries,
and epithelial cells. These cells secrete eight known hormones-growth
hormone (GH), prolactin (PRL), follicle-stimulating hormone (FSH),
luteinizing hormone (LH), thyroid-stimulating hormone (TSH),
adrenocorticotropic hormone (ACTH), melanocyte-stimulating hormone (MSH),
and endorphin (β-END). The epithelial cells that secrete these hormones are
organized into a glandular pattern and can be characterized on the basis of
their reaction with acid or basic dyes. In the human, most cells (52
percent) contain clear cytoplasm that does not stain with these dyes (chromophobes);
34 percent have cytoplasmic granules that stain with acid dyes (acidophils
or eosinophils); and 14 percent contain cytoplasmic granules that stain
with basic dyes (basophils). It has long been recognized that acidophils
secrete GH or PRL and that basophils secrete TSH, FSH, LH, or ACTH.
Elaborate classification schemes based on tinctorial properties of cells
after complex staining procedures have been proposed to further relate
epithelial cell structure to secretory function, but all these have failed.
Transmission
electron microscopy (TEM) demonstrates that these epithelial cells contain
large nuclei with dense chromatin and a single
nucleolus. The cytoplasm has a well-developed Golgi apparatus, abundant
rough endoplasmic reticulum, and large, dense core vesicles, which are sites
of hormone storage. The sequence of amino acids making up the
hormones is encoded in nuclear DNA. This information is in turn encoded in
messenger RNA (mRNA) by the process of transcription, and the mRNA conveys
it to ribosomes in the cytoplasm. A large body of evidence supports the
concept that amino acids are united in an orderly sequence on ribosomes to
form peptide prohormones-the process of translation. These large prohormones
in turn are inserted into the cisternae of the endoplasmic reticulum.
Membranes of the endoplasmic reticulum are pinched off to form lucent
vesicles. These vesicles transport the prohormone to the cis face of the
Golgi apparatus, and the prohormone is then passed through the Golgi
complex in a cis-trans sequence for modification by phosphorylation,
glycosylation, or sialylation as required for the assembly of a particular
pituitary hormone. In addition, posttranslational cleavage of peptide bonds
to form the final hormone product begins in the Golgi apparatus. Large,
electron-dense secretory granules containing the processed hormone are
assembled at the trans face of the Golgi
apparatus. These secretory granules serve as storage sites for the
hormones. On stimulation, the secretory granules migrate to the plasmalemma and unite with it, forming omega (Ω) figures. The contents of
the secretory granules are released by exocytosis.
Attempts were
made to devise a functional classification of adenohypophyseal cells on the
basis of their electron microscopic appearance (shape and size of cells and
distribution of vesicles) soon after these cells were first characterized
ultrastructurally. Functional cell types were labeled by identifying the
predominant cell type to appear after target organ ablation, then ascribing
an appropriate
tropic function to this cell type, and finally relating the cells to a
particular cell population in the normal pars distalis. For example,
corticotropes were identified by the appearance after adrenalectomy of "adenalectomy
cells" that resembled a specific population of cells in the normal
adenohypophysis. However, uncertainty remained when trying to distinguish
between some cell types on the basis of TEM appearance alone.
With the
advent of immunohistochemical procedures that stain hormones within cells. a
method was developed to more certainly identify functional cell types in the
adenohypophysis. At least six cell types have been identified (somatotropes,
lactotropes, gonadotropes. thyrotropes. corticotropes, and melanotropes).
The recent development of immunoelectron microscopy has added greatly to our
knowledge of the morphology of functional cell types in the adenohypophysis.
First. it has demonstrated that every pituitary hormone does not reside in
its own cell type. Whereas TSH is uniquely found in thyrotropes,
gonadotropes usually contain both FSH and LH. GH and PRL are usually found
in separate cells, but examples of mammosomatotropes have been found in
several species, including humans. Second, immunoelectron microscopy has
shown that the ultrastructural morphology of a given cell type varies with
the age of the animal, the hormonal milieu, and the functional state of the
cell. An example of this morphologic variation is found in the rat lactotrope. Three types of lactotropes have been described by
Kurosumi and colleagues. Type I cells are large and crescentic, with big
secretory granules (~500 nm) arrayed around the periphery of the cell. Type
II cells are smaller and are polygonal in shape. They are more granulated
than type I cells, but the granules are
small (~150 to 200 nm). Type III cells are small and polygonal and contain
only a few very small (~ 100 nm) secretory granules. In adult males. 46
percent of the lactotropes are type I, whereas in
adult females 91 percent are type I. Type III cells predominate in the
perinatal period but are almost absent in adulthood.
Functional
cell types in the pars distalis may not be immutable. Conversion of
somatotropes to mammotropes under the influence of estrogen has been shown
experimentally. In rats, propylthiouracil treatment has been observed to
cause transdifferentiation of somatotropes into thyrotropes. Pluripotent
cells capable of differentiating into corticotropes or thyrotropes have
been demonstrated by immunohistochemistry and hybrid histochemistry.
In most
species, the adenohypophysis is divided into three regions- the pars
tuberalis, pars intermedia, and pars distalis. The pars
tuberalis is applied to the surface of the median eminence and the upper
infundibular stem- rostral regions of the neurohypophysis. It is an extension
of the glandular pituitary that lies above the sella turcica, applied to the
base of the brain in the subarachnoid space. It is made up of epithelial
cells, fenestrated capillaries, and stromal cells. The epithelial cells have
been divided into pars tuberalis- specific cells, invasive cells, and
follicular cells. Pars tuberalis cells have a unique ultrastructural
appearance. They are spheroidal and contain glycogen granules and large
numbers of lysosomes, as well as a few small secretory granules at the
vascular pole. They immunostain weakly for TSH. The invasive cells appear
to be gonadotropes, because they contain secretory granules and stain with
antiserum to LH. Follicular cells are characterized by the presence of
microvilli and cilia at their apical surface. The role of the pars tuberalis
has not been firmly established, but studies suggest that it is
involved with maintenance of short-loop feedback in the regulation of gonadotropes.
The pars
intermedia, which is present in many species, is applied to the lower
portion of the lower infundibular stem and the infundibular process-caudal
regions of the neurohypophysis. It is made up of epithelial cells, with only
a few capillaries and stromal cells. Dopaminergic nerves are found in it and
terminate near glandular cells. Immunohistochemical and physiological
studies have demonstrated that its epithelial cells contain
α-MSH and
βendorphin. The pars intermedia is present in the human fetus and in
pregnant adult women but is absent in adult men and nonpregnant women.
However, in adult humans, basophilic epithelial cells are frequently found
invading the neural lobe, and immunohistochemical procedures demonstrate
many cells containing α-MSH closely apposed to the neurohypophysis. It
appears likely that these cells form a functional unit analogous to the pars
intermedia of other species.
The pars
distalis forms the bulk of the adenohypophysis. It is composed of epithelial
cells arranged in a glandular pattern, folliculostellate cells arranged in
a syncytium, and fenestrate capillaries. It contains no axon terminals.
Immunohistochemistry has revealed lactotropes, somatotropes, gonadotropes,
thyrotropes, corticotropes, and melanotropes in the pars distalis. Several
studies have demonstrated that lactotropes and somatotropes lie
predominantly in the lateral wings of the pars distalis, whereas
thyrotropes and
gonadotropes occupy its medial third-the "mucoid wedge," so called
because the secreted hormones (TSH, LH, FSH) are glycoproteins.
As this median zone is continuous with the pars tuberalis and contains
similar functional cell populations, it is also termed the zona tuberalis.
Corticotropes lie anteriorly in the mucoid wedge and over the surface of
the lateral wings. The secretory product is predominantly ACTH and
β-END. Corticomelanotropes lie posteriorly near the neural lobe, with a small
number scattered throughout the pars distalis. Their
secretory products are predominantly
α-MSH and
β-END.
Growth
hormone and prolactin are peptide hormones with similar structures. Growth
hormone affects the metabolic processes in all body tissues by stimulating
protein synthesis. Its actions are mediated by somatomedin-C (an
insulin-like growth factor), which is believed to be synthesized in the
liver. Prolactin stimulates protein synthesis (milk production) in the
estrogen-primed breast.
TSH, LH, and
FSH are glycoproteins and are each made up of two subunits: an
α chain and a
β chain. The
α chain, which is not biologically active, is common to the
three hormones. The β chain is unique to each hormone and is biologically
active. TSH supports thyroid structure and secretion. FSH supports growth of
the ovarian follicle and spermatogenesis. LH supports the corpus luteum and
estrogen and progesterone secretion in females and the Leydig cells and
testosterone production in males. ACTH is a peptide of 39 amino acids which
is secreted by corticotropes. α-MSH is a 13-amino-acid peptide secreted by
melanotropes. The structure of α-MSH is the same as that of the first 13
amino acids of ACTH. ACTH supports the structure and function of the adrenal
cortex to regulate the secretion of glucocorticoids.
α-MSH acts to disperse
melanophores in amphibian skin, but its role in humans is not well
established.
The secretion
of pituitary hormones from the pars distalis is regulated by several humoral
mechanisms. Hormone secretion from target
organs of the pituitary gland is maintained at appropriate levels by a
feedback mechanism. A decrease in the level of a circulating target hormone
is sensed by tropic adenohypophyseal cells, and the secretion of the
appropriate trophic hormone is increased. Other hormones and cytokines can
also alter pituitary function. For example, epinephrine released from the
adrenal medulla stimulates the release of ACTH, and interleukins, either
local or carried from distant sites, can also cause ACTH secretion. In
addition to these humoral mechanisms, neural mechanisms are also employed to
regulate pituitary function. The brain assists in the regulation of
pituitary function by releasing hormones from nerve terminals in the
pituitary.
The
Neurohypophysis
The
neurohypophysis is a diverticulum of the brain that makes its appearance in
the human early in fetal life (at between 10 and 14 mm crown-to-rump
length). The mature neurohypophysis is made up of axon terminals,
specialized glial cells, and blood vessels. Several features are common
throughout the neurohypophysis and serve to distinguish it from the
hypothalamus. The neurohypophysis contains no neuronal cell bodies-only
axons and axon terminals. Axons terminate in the perivascular space of
fenestrated capillaries, not on neurons or their processes. The
neurohypophysis lacks a blood-brain barrier. It regulates the function
of the adenohypophysis, which is applied to it.
The
neurohypophysis is subdivided into three regions on the basis of regional
morphologic specializations: (1) the median eminence, (2) the infundibular
stem, and (3) the neural lobe. The
median eminence and the paired lateral eminences together make up the tuber
cinereum, which is visible on the inferior surface of the brain lying
caudal to the optic chiasm and rostral to the paired mamillary bodies.
Because the median eminence forms the
funnel-shaped floor of the third ventricle, it is also called the
infundibulum. It is the rostral region of the neurohypophysis. The
infundibular stem is the neural portion of the pituitary stalk.
The neural
lobe (also called the infundibular process) is the caudal region of the
neurohypophysis. The classification of the neurohypophysis into these three
portions stresses the observation that it is a diverticulum of brain and is
distinct from the hypothalamus with which it is contiguous.
The
infundibulum, the floor of the third ventricle, is separated into an
ependymal layer, an internal zone, and an external (or palisade) zone. Its ependymal layer is made up of specialized epithelial cells.
They are united by zonulae occludens (tight junctions), which inhibit the
passive exchange of materials between the third ventricle and the
interstitial fluid of the infundibulum. These cells lack cilia at their
ventricular (apical) surface, which instead is characterized by the presence
of large blebs as well as numerous smaller microvilli. Some of these ependymal cells are stretched ("tanycytes"), with their apical surface
facing ventricular fluid and their basilar processes terminating in the
perivascular space of fenestrated capillaries on the surface of the median
eminence. Such cells do not resemble the ependymal cells lining the walls of
the third ventricle, which are cuboidal, have cilia at their apical surface,
lack apical blebs, and are united by gap junctions and desmosomes. The
ependymal cells that line the infundibulum resemble the ependymal cells
lining the rudimentary neural tube during embryonic development.
The internal
zone of the median eminence is made up of axons of the supraopticohypophyseal
tract, which originate in the hypothalamic supraoptic and paraventricular
nuclei and pass through the median eminence to terminate in the neural lobe.
The supraopticohypophyseal tract is selectively stained by acid fuchsin
techniques in histologic studies and by antibodies to vasopressin and
oxytocin (OT) for immunohistochemical studies. Its axons are typically
large (magnocellular). These magnocellular axons show dense core vesicles
(150 to 300 nm in diameter) on TEM. Small (parvicellular) fibers and terminals containing ACTH,
α-MSH, and
β-END also lie in the internal zone.
In addition to peptidergic fibers, noradrenergic fibers and terminals have
been demonstrated in the internal zone by formaldehyde fluorescence
techniques. These fibers are believed to originate outside the hypothalamus
in the brain
stem and to represent the terminus of the ascending reticuloinfundibular
tract.
The external
zone of the median eminence is made up of glial cells, axons, and axon
terminals. The axon terminals lie in the perivascular space of fenestrated
capillaries. They are smaller than the terminals in the neural lobe and are
part of the parvicellular neurosecretory system. Early investigators noted
that these terminals could be separated into two classes based on the types
of vesicles they contain: (1) terminals containing only small (50 nm),
lucent vesicles and (2) terminals containing both small, lucent synaptic
vesicles and large, granular vesicles (~100 nm). Subsequent studies have
shown that the small, lucent vesicles contain synapsin and synaptophysin and
thus resemble the synaptic vesicles found in terminals throughout the brain.
Immunoelectron microscopic studies have localized peptide neurosecretory
hormones in the large granular vesicles.
The
disposition of terminals in the external zone has not been well
characterized in humans but has been detailed in other mammals. The
external zone has long been recognized as the site where the dopaminergic
tuberoinfundibular tract terminates. It has also been recognized as a site
where releasing hormones are secreted by hypothalamic neurons. At one time,
a strict distinction was thought to exist between hypothalamic parvicellular
neurons, which projected to the median eminence and released catecholamines
(aminergic neurons), and hypothalamic parvicellular neurons, which projected
to the median eminence and released hypothalamic hypophysiotropic hormones
(peptidergic neurons). It was assumed that each terminal contained only one
biologically active substance that was destined to be carried from the
median eminence to the pars distalis to regulate its function. This
assumption has proven to be false; the discovery of colocalization of
peptides and transmitters in terminals of the median eminence has blurred
the distinction between aminergic and peptidergic neurons.
Terminals
containing thyrotropin releasing hormone (TRH) reside in the external zone
of the median eminence. TRH stimulates the release of TSH from thyrotropes.
Terminals that contain corticotropin releasing hormone (CRH) also reside
there, but many of these terminals co-store arginine vasopressin (AVP). Some
costore angiotensin (ANGII), and some neurons contain all three hormones.
Each hormone acts to stimulate the release of ACTH from corticotropes. The
actions of CRH and AVP are not only additive but synergistic. The terminals
that contain TRH, CRH, somatostatin (SRIF), or growth hormone releasing
hormone (GRH) all lie in the medial third of the external plexus in the rat.
Terminals that contain gonadotropin releasing hormone (GnRH) are present in
the external zone; but, in the rat, they are located in the lateral thirds
of the median eminence as seen in coronal section. The distribution of
parvicellular terminals in the human median eminence has not been
characterized.
Dopaminergic
terminals are also present in the external zone of the median eminence. They
have been identified by their characteristic fluorescence after exposure to
formaldehyde vapours or to gyloxylic acid or by the immunohistochemical
identification of tyrosine hydroxylase in them. Tyrosine hydroxylase-like
(TH-li) terminals are presumed to be dopaminergic. Dopamine inhibits the
release of PRL from lactotropes. Neurotensin (NT), galanin (GAL), and gamma
amino butyric acid (GABA), have all been colocalized in TH-li terminals in
the rat median eminence.
The
infundibular stem lies between the infundibulum and the infundibular
process. It is characterized by the presence of axons of the
supraopticohypophyseal tract. The
infundibular process (neural lobe) is the terminus of the
supraopticohypophyseal tract, the axons of which terminate in the
perivascular space of neural lobe capillaries. Some parvicellular systems
also terminate in the neural lobe in the human.
The Portal
System: Transfer of Information from Neurohypophysis to Adenohypophysis
In the
somatic efferent system, information is carried as a pattern of neural
impulses by ventral horn cells that are the final common pathway to striated
muscle cells. The pattern of impulses carried along the ventral horn cells
is determined by segmental and suprasegmental (descending) input across
chemical synapses, and the information reaches the target (muscle) cell
through a chemical synapse at the myoneural junction. The central nervous
system employs the mechanism of neurotransmission to cause the effector organ
(striated muscle) to exert a force over a distance with considerable
precision.
In the
visceral efferent system, a similar arrangement is found. Suprasegmental and
reflex (segmental) inputs converge on cell bodies in the intermediolateral
cell column (or in visceral efferent cranial nerve nuclei), the axons of
which serve as the final common pathway to the effector organ. In the case
of cardiovascular regulation, the effector organ is again a muscle cell
(smooth or cardiac). The visceral efferent system also regulates glandular
secretion-for example, gastrointestinal, salivary, lacrimal, pineal and
choroid plexus secretions. In this system, postganglionic neurons make
synaptic contact with secretory epithelial cells. In the case of the adrenal
medulla, preganglionic neurons make synaptoid contact with cells that
originated in the neural crest and have been modified into secretory
epithelium. The secretory activity of glandular cells is regulated by the
central nervous system in a manner analogous to the regulation of muscle
cells. Descending (suprasegmental) and reflex (segmental) inputs are
integrated into the final common pathway to regulate the performance of
chemical work by secretory epithelial cells (as opposed to mechanical work
by muscle cells).
The third
efferent system-the neuroendocrine system-may be viewed in an analogous
manner. In this system, the effector organ is a secretory epithelial organ
that performs the chemical work of synthesizing and secreting hormones.
These secretory epithelial cells, like muscle cells or exocrine glandular
cells, lie at some distance from the central neural elements that control
their function. However, the final common pathway to these endocrine cells
is not axonal but vascular, and the information is carried to the pituitary
(effector) cells by neurohormones, which are released in a pulsatile pattern
from neurohypophyseal axon terminals by the process of neurosecretion into
restricted vascular channels that pass to the adenohypophysis.
Since the
blood vessels in the pituitary gland are the final common pathway from the
terminals in the neurohypophysis to the effector cells in the
adenohypophysis, their anatomy merits attention. The three neurohypophyseal
regions share a common capillary bed. It is supplied rostrally at the
median eminence by the superior hypophyseal arteries, which arise from the
intracranial internal carotid arteries, the proximal (A1) segments of the
anterior cerebral arteries, and the posterior communicating arteries; and it
is supplied caudally at the neural lobe by the inferior hypophyseal
arteries. In several species there is a third source of arterial supply,
variously called the trabecular artery, the loral artery, the anterior
hypophyseal artery, and the artery to the infundibular stem, depending on
the species studied. As this vessel supplies the infundibular stem and
arises along the course of the internal carotid artery between the superior
and inferior hypophyseal arteries, the name middle hypophyseal artery seems
appropriate.
The neurohypophyseal capillary bed is
specialized at its rostral pole into a complex median eminence (primary)
capillary plexus. This plexus is subdivided into an external (mantle) plexus
and an internal plexus of complex capillary loops and coils. The external
plexus lies on the median eminence surface, interposed between the median
eminence and the pars tuberalis. Its fenestrated capillaries lie in close
proximity to axon terminals in the external zone of the median eminence. The
internal plexus arises from the external plexus, and its complex vascular
formations penetrate the median eminence neuropil. Some (short) capillary
loops penetrate only through the external zone, whereas other (long)
capillary loops penetrate through the external and internal zones to the subependymal region before returning to the surface of the median eminence.
The
neurohypophysis is drained rostrally by a series of fenestrated portal
vessels that are interposed between the median eminence primary plexus and
the pars distalis secondary plexus. These portal vessels drain both the
external and the internal plexus. Capillary connections between the median
eminence and the pars distalis are also ample. In addition, drainage routes
from the median eminence to the hypothalamus and to the veins at the
cerebral base have been demonstrated in several species. The rostral region
of the neurohypophysis has limited drainage to the general circulation, but
ample local drainage to the pars distalis.
The
neurohypophysis is drained caudally (at the level of the neural lobe) by
paired posterior hypophyseal veins, which course parallel to the inferior
hypophyseal arteries and drain to the posterior cavernous sinus lateral to
the midline. In addition, capillary connections unite the neural lobe with
the adjacent pars distalis, and, in some species (for example, the rat). a
series of short portal vessels also unite the neural lobe with the pars
distalis. The caudal region of the neurohypophysis has ample drainage to the
general circulation but somewhat limited local drainage to the adjacent pars
distalis.
The
adenohypophysis does not receive a direct arterial supply; the blood
entering it passes first through the neurohypophysis. The venous drainage of
the adenohypophysis is from the pars distalis to the adjacent cavernous
sinus. The primary drainage routes are through the same posterior
hypophyseal veins that drain the neurohypophysis. These veins are Y
-shaped, with one arm draining the caudal neurohypophysis and the other
draining the caudal pars distalis; the arms unite into a common stem that
drains into the posterior cavernous sinus. In addition, small lateral
hypophyseal veins course from the lateral wings of the pars distalis into
the lateral cavernous sinus.
The direction
of blood flow in the pituitary gland has been deduced from histologic
examination of the gland after sectioning of the stalk and from direct
observations of the flow of blood from the median eminence down the anterior
surface of the pituitary stalk to the pars distalis in living animals. The
pattern of blood flow on the surface of the entire gland has been recorded. Blood enters the neurohypophysis at each end (the median
eminence and the neural lobe) almost simultaneously. However, because the
inferior hypophyseal arteries lie closer to the heart than the superior
hypophyseal arteries, blood enters the neural lobe just prior to entering
the median eminence. Within the neurohypophysis, blood flow between
different regions occurs. It flows retrograde from the neural lobe into the
lower infundibular stem and anterograde from the median eminence into the
upper infundibular stem. The infundibular stem is thus a watershed zone,
receiving blood from both the median eminence and the neural lobe. The site
in the infundibular stem where the ascending and descending wave fronts
meet varies. Rostrally the median eminence drains rapidly to the pars
distalis. Caudally the neural lobe and infundibular stem drain to the
cavernous sinus through posterior hypophyseal veins. Some blood does cross
from the neural lobe into a small zone of the adjacent pars distalis. The
bulk of the pars distalis, however, receives its blood from the median
eminence. Blood passes down the pituitary stalk into the medial third of the
pars distalis and courses into the lateral wings. A small region in the
lateral wings of the pars distalis drains to the lateral cavernous sinus
through lateral hypophyseal veins. Most of the blood sweeps dorsomedially to
the junction of the pars distalis and neural lobe, where it drains into the
Y-shaped pituitary veins, which in turn drain to the posterior cavernous
sinus. Sectioning of the stalk markedly alters the pattern of blood flow. No
blood enters the pars distalis through portal routes from the median
eminence. The small territory of
the pars distalis supplied from the neural lobe diminishes if the blood
pressure falls.
Although the
physiology of pituitary blood flow has come under close scrutiny, a
composite picture cannot as yet be presented. Pars distalis blood flow in
the rat and dog is about 70 cm3/100 g per minute, and neurohypophyseal
blood flow in the sheep is much higher-about 450 cm3/100 g per minute. This
flow is about 8 times the cortical blood flow and 10 times the hypothalamic
blood flow in the same animals. Flow in the median eminence does not differ
from that in the neural lobe. Neurohypophyseal blood flow is autoregulated
and decreases with hypocarbia. In these respects it is similar to cerebral
blood flow, a finding that should not be unexpected in a brain
diverticulum. As neurosecretory cells terminate on median eminence
capillaries that drain to the pars distalis via portal routes and on
capillaries in the infundibular stem and neural lobe that drain to the
adjacent pars distalis, the entire
neurohypophyseal capillary bed may be viewed as the final common pathway to
the pars distalis. The vascular arrangements in the pituitary gland assure
the delivery of neurosecretions from the neurohypophysis to the
adenohypophysis over local routes at high rates of flow.
Neurosecretion into neurohypophyseal vessels provides the mechanism by which
the brain controls adenohypophyseal function. The Scharrers proposed the
concept of neurosecretion. They suggested that some neurons could function
as glandular cells, synthesizing and releasing hormones. Subsequently, the
hormones AVP and oxytocin (OT) were isolated from the neural lobe and the
hypothalamic supraoptic and paraventricular nuclei. With the demonstration
that OT and AVP are synthesized in these hypothalamic regions, transported
by axoplasmic flow through fibers in the supraopticohypophyseal tract to
axon terminals in the neural lobe, and released under appropriate
physiological conditions, the concept of neurosecretion was established and
the means by which the brain controls neural lobe function was clarified. In 1947, Green and Harris demonstrated that the pars distalis
lacks axon terminals, and they suggested that a neurovascular link between
the median eminence and pars distalis controlled anterior pituitary
function. They postulated that chemical messengers are released from axon
terminals that lie in the perivascular space of capillaries in the median
eminence, enter these capillaries, and are transported by restricted portal
routes to target cells in the pars distalis.
Hormones were
found in the median eminence and medial basilar hypothalamus by bioassay;
and, they regulated adenohypophyseal function. Several of these peptide
hypothalamic hypophysiotropic hormones have been isolated, sequenced, and
synthesized. GnRH stimulates the secretion of both FSH and LH by
gonadotropes. TRH stimulates the release of TSH by thyrotropes. CRH
stimulates the release of ACTH by corticotropes. GRH stimulates the release
of growth hormone from somatotropes, whereas somatostatin SRIF inhibits it.
In addition, evidence is accumulating that dopamine is a physiological
prolactin-inhibiting factor.
In the
somatic efferent system, specificity of response is assured by the close
proximity of the axon terminal to the muscle cell, the isolation of the
synapse at the myoneural junction, and the specificity of receptor sites on
the muscle cell membrane. In the pars distalis, epithelial cells are
virtually bathed in blood containing a host of
hypothalamic releasing and inhibiting hormones. These hormones interact with
specific receptors on the cell surface of target pituitary epithelial cells
to activate a cascade of intracellular second messengers that stimulate or
inhibit the synthesis and release of adenohypophyseal hormones. There is
accumulating evidence that the releasing hormones GnRH and TRH stimulate
intracellular cAMP production in gonadotropes and thyrotropes, and that the
inhibiting hormones somatostatin and dopamine inhibit cAMP production in somatotropes and lactotropes. Increased production of ACTH by corticotropes
depends on the activation of both cAMP and the calcium-calmodulin system.
Stimulated release of PRL from lactotropes depends on the activation of
phospholipid hydrolysis. The brain evokes a specific pituitary hormone
response by releasing specific hypothalamic stimulating and inhibiting
hormones, which interact with specific receptors on pituitary
target cells.
In its
simplest form, this schema involves three assumptions: (1) that the
specificity of receptors at the adenohypophyseal cell surface is absolute,
(2) that only one stimulating and/or releasing hormone governs the secretory
activity of each functional pituitary cell type (i.e., that each
adenohypophyseal cell carries only one population of surface receptors), and
(3) that a single releasing hormone
releases only one pituitary hormone. Regarding the first assumption,
however, the specificity of hypothalamic hypophysiotropic hormones is not
absolute. TRH administration stimulates the release of PRL as well as TSH,
although the role of TRH in the physiologic control of PRL secretion has not
as yet been established. Somatostatin inhibits the synthesis and release of
hormones other than growth hormone. In certain pathologic conditions, for
example adenoma formation, cellular receptors apparently lose their
specificity. In some acromegalics, growth hormone secretion is stimulated by
TRH infusion and is inhibited by the administration of dopamine agonists.
As for the second assumption, some
adenohypophyseal cells respond to two or more hypothalamic peptidergic
hormones.
Pituitary
ACTH secretion is stimulated by AVP as well as CRH. Although the molar
response of corticotropes to CRH is much stronger than to AVP, the effect of
the two hormones combined is not simply additive but is synergistic. In
addition, ACTH release can be increased by the levels of epinephrine that
are reached under conditions of stress, via the stimulation of
β-adrenergic
receptors on corticotropes. Corticotropes have three receptor types (at
least) on their cell surface. Each is activated by a specific agonist and
in turn activates a cascade of second messengers.
Regarding the
third assumption, although receptor specificity on the adenohypophyseal cell
surface is a mechanism that would appear to guarantee that a specific
pituitary hormone is released in response to a
specific brain hormone, CRH apparently stimulates both the
secretion of ACTH from corticotropes and the secretion of MSH from
melanotropes in the rat pituitary. The explanation for this finding lies
in the fact that the two cell lines carry out different posttranslational
processing on the same prohormone. Both corticotropes and melanotropes
translate the same prohormone (proopiomelanocortin) from mRNA. Both cleave
this 31,000-dalton prohormone into a precursor hormone containing 130 amino
acids. Corticotropes then cleave this precursor to form ACTH (amino acids 1
to 39) and β-END, whereas in melanotropes, ACTH is further cleaved into
α-MSH
(amino acids 1 to 13) and corticotropin-like intermediate peptide (CLlP).
The
specificity of response may be aided by an endocrinotopic segregation of
nerve terminals. Dopaminergic systems (from the arcuate
nucleus) terminate in the external zone, principally in the
lateral third. Norepinephrine-containing terminals of neurons arising in the
brain stem terminate in the medial third of the median eminence, principally
in the internal zone. CRH projections terminate in the medial third
(internal and external zone), and AVP systems pass through the median
eminence internal zone to the neural lobe but also terminate in the external
zone in the medial third. Somatostatin-containing fibers terminate diffusely
in the medial third, and TRH-containing fibers terminate in the external
zone of the middle third. Therefore, the lateral thirds of the median
eminence contain neurosecretory and catecholamine terminals (producing GnRH
and dopamine) that are involved in regulating reproduction through the
regulation of FSH, LH, and PRL secretion. The medial third produces the
releasing hormones that are involved in
regulating metabolism through GH and TSH secretion (somatostatin, GRH, and
TRH) and in mediating the organism's response to stress through ACTH
secretion (CRH and AVP). Portal vessels are
so disposed as to carry hypothalamic hormones from the lateral regions of
the median eminence to the lateral wings of the pars distalis and from the
medial third of the median eminence to the mucoid wedge of the pars
distalis.
Vascular
elements need not be interposed between the neural terminal and
adenohypophyseal secretory cells. The dopaminergic tuberohypophyseal tract
terminates along the ventral surface of the infundibular
stem and process, near melanotropes in the adjacent pars distalis whose
adenyl cyclase and secretory activity are negatively coupled to dopamine
concentration. In some regions, these
adenohypophyseal cells invade the neural lobe. The proximity of nerve
terminals to melanotropes provides another mechanism for specific control of
adenohypophyseal function by the brain.
The
specificity of the receptors at the adenohypophyseal cell surface for
corresponding peptidergic hypothalamic hormones, the genetically determined
translational pathways of (pro-) hormone synthesis, and the variations in
post-translational processing of prohormones are the key mechanisms in
assuring a specific adenohypophyseal response to neurohumoral instructions
from the brain. The close relation of melanotropes to parvicellular
terminals, which
obviates the need for vascular transport of humoral signals, is a fourth
such mechanism. The possibility that specific regions in the median
eminence "project" by portal vessels to corresponding pituitary regions
adds another potential mechanism of neural control of anterior pituitary
function, and is presently being investigated (Table-1).
TABLE-1
Neurosecretory Systems that Regulate Pituitary Function |
RH/IH* |
Pituitary Hormone |
Hypothalamic Origin |
Neurohypophyseal Terminus |
Location of pituitary target cells |
Hypothalamic Neural Projection |
Reproductive Systems |
GnRH |
FSH |
POA |
ME (lateral third) |
PT |
Amygdala |
|
LH |
|
|
PD (mucoid wedge) |
Mesencephalon |
Dopamine |
PRL |
TN |
ME (lateral third) |
PD (lateral wings) |
? |
Metabolic Svstems |
TRH |
TSH |
PVN |
ME (medial third) |
PT |
? |
|
|
|
Neural lobe |
PD (mucoid wedge) |
? |
SOM |
GH |
PVN |
ME (diffuse) |
PD (lateral wings) |
|
CRH |
ACTH |
PVN |
ME (medial third) |
Central; anterior |
? |
AVP |
ACTH |
PVN |
ME (medial third) |
Central; anterior |
Medulla |
AVP |
ACTH |
SON |
NL (central) |
Central; anterior |
? |
|
|
PVN |
|
|
|
OX |
?ACTH |
PVN |
NL (peripheral, next to pars distalis) |
Next to neural lobe |
Medulla
Spinal cord |
Dopamine |
MSH |
TN |
NL (next to pars distalis) |
Near neural terminals |
? |
* RH =
releasing hormone, IH = inhibiting hormone. POA =
preoptic area, TN = tuberal nuclei, PVN = paraventricular nuclei, SON =
supraoptic nuclei, ME = median eminence, NL = neural lobe.* PT =
pars tuberalis, PO = pars distalis.
Surgical
Correlates of Pituitary Anatomy
This anatomic
survey has constructed a pituitary gland composed of a neurohypophysis
(median eminence, infundibular stem, and neural lobe) and an adenohypophysis
(pars tuberalis, pars intermedia, and pars distalis). The proposed model is
quite different in some respects from the traditional teaching that the
pituitary gland lies in the sella turcica and is bounded superiorly by the
diaphragma sellae; that the median eminence is part of the hypothalamus;
and that the pars tuberalis can be dismissed. That version arose from the
habit of anatomists and pathologists of dividing the pituitary stalk when
removing the brain from the skull thus keeping the median eminence and pars
tuberalis with the brain and leaving a "pituitary gland" consisting of the
pars distalis and neural lobe in the sella turcica. The traditional
pituitary gland thus represents only half of the pituitary as defined here.
The model
presented here raises two points of surgical interest. First, total
hypophysectomy by the transphenoidal route becomes a difficult and dangerous
task that involves removing the median eminence and pars tuberalis. Because
infarction of the median eminence and pars tuberalis does not occur (in
monkeys and rats) following either sectioning of the stalk or removal of the
pars distalis and neural lobe, functioning tissue capable of secreting FSH
and LH is left behind when only the pars distalis is removed. Indeed, in
the rat, growth of this tissue occurs after hypophysectomy, with an
increase in the number and size of functioning gonadotropes. to Second,
knowledge of the distribution of cell types in the adenohypophysis makes it
possible to predict the sites of small functioning
pituitary tumors. Tumors that secrete GH or PRL will be found in the
lateral wings of the pars distalis, whereas
tumors secreting TSH or gonadotropins will be found more medially in the
mucoid wedge or above the diaphragma sellae in the pars tuberalis. Tumors
that secrete α-MSH or ,l3-endorphin may be expected to be closely apposed to
the neural lobe, whereas ACTHsecreting tumors may be expected to begin
anteriorly and inferiorly near the median plane.
Several
points of surgical relevance also become apparent when one considers the
portal system. (1) When vasopressors are infused during hemorrhagic shock to
raise mean arterial blood pressure and restore cerebral blood flow, they
will have no effect on cerebral vascular resistance because of the
blood-brain barrier. However, in the pituitary gland, where there is no
blood-brain barrier, pressor agents can reach the smooth muscle cells of
resistance arterioles, causing them to constrict and decrease pituitary
blood flow, with the result that pituitary infarction can occur even while
cerebral blood flow is being restored. This mechanism may be the cause of
Sheehan' s syndrome of postpartum pituitary necrosis. (2) Because the
superior hypophyseal arteries that vascularize the median eminence and
medial basilar hypothalamus lie in the subarachnoid space, subarachnoid
hemorrhage with spasm of the vessels of the circle of Willis may be expected
to reduce pituitary blood flow and alter pituitary function. This scenario
will be masked by the administration of glucocorticoids. (3) Because the
venous drainage routes from the pars distalis to the cavernous sinus lie
lateral to the midline, it should be possible to lateralize small
secretory pituitary tumors that are poorly visualized by radiologic studies
by performing selective bilateral inferior petrosal sinus catheterization
and taking blood samples for determination of hormone levels. (4) The venous
drainage routes to the cerebral base from the
median eminence are apparently adequate to prevent venous infarction of the
median eminence after sectioning of the stalk or removal of the neural lobe
and pars distalis. Therefore, if the pituitary gland in the sella has
undergone destruction by a neoplasm or aneurysm or by traumatic stalk
sectioning, it should be feasible to transplant the pituitary to sites
beneath the median eminence, as only the portal vascular connections between
the graft and the preserved median eminence need be re-established not
neural connections.
Hypothalamic
Anatomy
Endocrine
"Motor" Neurons in the Hypothalamus
The chemical
synthesis of peptide hypothalamic hormones has made it possible to identify
them by immunohistochemistry in neurosecretory cells whose cell bodies lie
in the hypothalamus and whose terminals lie in the median eminence. Neural
hormones are synthesized in the neuronal soma (on rough endoplasmic
reticulum) by the same steps that produce pituitary peptides in
adenohypophyseal cells. The hormones are packaged in large, granular
vesicles and transported by axoplasmic flow to the axon terminal lying in
the perivascular space of a fenestrated capillary in the neurohypophysis
where they are released by exocytosis under appropriate physiological
conditions. Recent evidence suggests that the hormone translated from
mRNA is frequently a prohormone, and that the cleavage of this prohormone
occurs during axoplasmic
transport.
In the rat,
parvicellular neurosecretory neurons terminate in the rostral
region of the neurohypophysis (the median eminence), and their
neurosecretions regulate pars distalis function. In the human, the terminal
sites of the parvicellular system are more widespread, with some lying in
the neural lobe as well as the ones in the median eminence. A typical CRH-li
parvicellular neuron in the rat hypothalamus is bipolar in shape, with a
fusiform cell body 20 to 28 µm long by 10 to 12
µm wide. The nucleus is in
folded and contains a prominent nucleolus. The Golgi apparatus and rough
endoplasmic reticulum are prominent. Immunoreactive CRH is present on
ribosomes in the cell body and in dendrites as well as in granular vesicles
in the cell body and axons.
Hypophysiotropic neurons have been identified by combining retrograde
tracing techniques with immunohistochemistry.
Most of the work has been carried out in experimental animals such
as the rat. so extrapolation to humans may be incorrect. For
the most part, parvicellular neurosecretory cells are localized in nuclear
clusters. GRH-li neurons are present in the arcuate nucleus, as are neurons
that contain the prolactin inhibiting hormone dopamine. In humans, GnRH-li
neurons are found aggregated anterior to the median eminence just beneath
the arcuate nucleus as well as in the preoptic area. Hypophysiotropic SRIF-li
neurons and TRH-li neurons are present in the anterior periventricular
nucleus. CRH-li
neurons are present in the paraventricular nucleus.
The anatomy
of this nucleus is complex. It is composed both of magnocellular neurons
that contain oxytocin or vasopressin and project to the neural lobe and of
parvicellular neurons that contain CRH and project to the median eminence.
These neuronal populations are
segregated in the nucleus such that each region has a unique population of
immunohistochemically identifiable cells and a unique pattern of input and
efferent projection.
Parvicellular
neurosecretory neurons synthesize and co-store hormones other than the
classic releasing and inhibiting hormones, A population of CRH-li neurons
co-store AVP and/or ANGII; another population of CRH-li neurons co-store vasoactive
intestinal peptide (VIP) and metenkephalin; another costores
neurotensin, and yet another costores galanin. Presumptive dopaminergic (THli)
neurons can also be subdivided into different populations characterized by
unique patterns of costored hormones. Immunoreactive glutamic
acid decarboxylase (GAD) has been demonstrated in some TH-li neurons,
suggesting that GABA and dopamine are costored in the same neurons. GRF.
neurotensin, choline acetyltransferase, and galanin have been colocalized
in TH-li neurons by
immunohistochemistry in different patterns. The significance of the
hormonal profiles that define specific functional populations of
hypothalamic neurosecretory cells is not well understood. Perhaps hormones
and transmitters that are colocalized with releasing and inhibiting hormones
act by autocrine or paracrine mechanisms to optimize the effect of
neurohormone release.
Descending
Fiber Systems to the Neurohypophysis
Neuronal cell
bodies containing immunoreactive CRH in the parvicellular portion of the
paraventricular nucleus project laterally from the nucleus along with the
projections of the magnocellular AVP and OT neurons to enter the lateral
retrochiasmatic area. This CRH projection then, passes posteromedially to
enter the median eminence from the anterolateral preoptic region.
Hypophysiotropic TRH-li neurons occupy both the anterior periventricular
nucleus and the periventricular zone of the paraventricular nucleus in the
rat. The largest population of immunoreactive neurons is found in the
parvicellular division of the paraventricular
nucleus. These neurons take a descending periventricular course to the
median eminence. SRIF-li neurons that project to the median eminence also
lie in the anterior periventricular nucleus and project to the median
eminence by periventricular routes. TH-li neurons lie in the dorsomedial
region of the arcuate nucleus, and GRH-li neurons lie in its ventrolateral aspect. These neurons too project to the median eminence. Taken
together. the projections of CRH neurons from the paraventricular nucleus,
the projections of SRIF-li neurons and TRH-li neurons from the anterior periventricular nucleus. and the projections of the TH-li neurons and GRH-li
neurons from the arcuate nucleus constitute the tuberoinfundibular tract
GnRH-containing perikarya are concentrated in the medial preoptic area
rostral and dorsal to the chiasm in many of the commonly studied
experimental animals with an estrus cycle. Menstruating primates, as noted,
have a different distribution of GnRH-li neurons, with an aggregation of
cells anterior and inferior to the arcuate nucleus. GnRH-containing cells in
the preoptic area project through the preopticoinfundibular tract to
terminate in the median eminence.
In addition
to the parvicellular peptidergic system, which secretes hypothalamic
releasing and inhibiting hormones, there is a second peptidergic system in
the hypothalamus. The cell bodies of this system, which are located
predominantly in the arcuate nucleus, contain the processed products of
pro-opiomelanocortinACTH, β-lipotropin (β-LPH),
α-MSH, and
β-endorphin.
The system projects to the median eminence. In addition, ACTHcontaining
projections from the arcuate nucleus to the parvicellular regions of the
paraventricular nucleus have been demonstrated, as have projections
containing α-MSH,
β-endorphin, ACTH, and
β-LPH to hypothalamic and extrahypothalamic brain areas.
Surgical
Correlates of Hypothalamic Anatomy
If the
anatomic findings described here can be extrapolated to humans, they have
several implications that are relevant to surgery. (1) Tumors of the medial
sphenoid ridge may alter anterior pituitary function without direct
pituitary involvement by compressing hypothalamic-hypophysiotropic
pathways. (2) Because pathways projecting from the paraventricular nucleus
to the median eminence lie close to the third ventricle, they will be
susceptible to injury by surgical procedures carried out in the third
ventricle, as will the periventricular cell bodies. If the median eminence
or pars distalis has been compromised by a tumor or by an operation to
remove the tumor, damage to the periventricular hypothalamus will be
masked, as is damage to the corticospinal tract or ventral horn cells when
the target muscle is diseased or injured. (3) The anterior surgical approach
to the third ventricle through the lamina terminalis must be kept strictly
in the midline to avoid damaging fibers passing to the median eminence and
neural lobe. (4) Damage to small vessels entering the anterior perforated
space during aneurysm dissection will damage pituitary function as well as
autonomic function and consciousness. The menstrual cycle and reproductive
function in women of child-bearing age would appear to be particularly at
risk. (5) Hydrocephalus with stretching of periventricular fibers may alter
pituitary function. (6) The periventricular location of these
hypophysiotropic fiber systems and the recovery of hypothalamic hormones
from cerebrospinal fluid suggest that transplantation of pituitary tissue
into the third ventricle, as well as beneath the median eminence, is
feasible.
Extrasegmental Input to the Hypothalamus
The adenohypophyseal cells are viewed as being analogous to a motor
organ such as skeletal muscle; the neurohypophyseal capillaries and the
portal system are viewed as the final common pathway to these cells; and the
neurosecretory cells and their fiber tracts containing hypophysiotropic
peptides, which originate in the periventricular region of the hypothalamus
and preoptic area and terminate near neurohypophyseal capillaries, are
presented as analogous to segmental ventral horn cells. The tasks remaining
are (1) to determine the extrasegmental neural inputs to hypothalamic
neurosecretory cell groups, to clarify the mechanisms by
which information converging on endocrine motor units is integrated; and (2)
to determine the pathways of neural output from these hypothalamic cell
groups, to clarify the mechanisms by which autonomic, motor, and mental
activities are correlated with neuroendocrine function.
The role of
descending suprasegmental input from the temporal lobes in the control of
reproductive function has been demonstrated, but the anatomy of
suprasegmental control of GnRH remains to be clarified. At the segmental
level, we know that in the rabbit, preoptic stimulation in the region
containing GnRH cell bodies elicits GnRH release into portal vessels,
resulting in elevation of plasma LH levels and ovulation. We also know that
lesions of suprasegmental neurons in the amygdala or of their efferent
projections in the stria terminalis (which terminates in the sexually
dimorphic nucleus of the stria terminalis in the preoptic area) alter the
estrus cycle in the rat. In primates, the relationship between the medial
temporal lobes and reproductive function is not clear. No change in
neuroendocrine function was reported in adult female monkeys that had
undergone bilateral anterior temporal lobectomy. However, alterations in
the control of LH secretion have been reported in humans with temporal lobe
epilepsy. The mechanism by which temporal lobe output modifies the release
of FSH and/or LH from the pituitary gland awaits clarification of the
descending input from the temporal lobe to the GnRH cells scattered in the preoptic area and (in humans) clustered beneath the arcuate nucleus.
There is a
significant input from the amygdala and hippocampal formation to both the
magnocellular and the parvicellular regions of the paraventricular nucleus,
but it is not direct. The input to the parvicellular regions is via a relay
in the bed nucleus of the stria terminalis. Input from the amygdala reaches
the magnocellular regions of the paraventricular nucleus and the supraoptic
nucleus through its projection to the ventromedial nucleus, thence to the
dorsomedial nucleus and on to the regions where magnocellular
neurosecretory neurons reside. In addition, projections from the septum
(which in turn receives hippocampal input) to the paraventricular nucleus
have been found. Stimulation of the dorsal hippocampus or of the septum
inhibits firing in neurons that lie in the paraventricular nucleus and
project to the median eminence. Excision of the hippocampi or sectioning of
the fornices results in an increase in plasma cortisol levels. It seems
reasonable to conclude that descending input from the temporal lobes
inhibits ACTH release from corticotropes in the pars distalis via
inhibition of the release of CRH from CRH-li neurons in the paraventricular
nucleus.
The response
to hypotension provides a model that permits insight into the mechanisms by
which neuroendocrine and visceral responses are integrated by ascending infrasegmental
input. Except in the case of feedback mechanisms, it is unusual for the
organism to respond to a stimulus from the internal or external milieu by
secreting only one pituitary hormone. For instance, in hypotensive shock, ACTH,
β-END,
and AVP are all released from the pituitary gland, and compensatory
adjustments are also made by the visceral efferent (autonomic) system.
Hypotension
causes an increase in neural traffic over the glossopharyngeal nerves, with
increased input to the tractus and nucleus solitarius. Connections between
the nucleus solitarius and the dorsal vagal motor nucleus have been found,
as have connections between the dorsal motor nucleus and the lateral
reticular nucleus. Ascending noradrenergic systems originating in the
dorsal motor nucleus and in the lateral reticular nucleus have been
demonstrated in the rat by the use of formaldehyde fluorescence techniques.
Neurons from the noradrenergic (A2) region of the dorsal motor
nuclei terminate in the parvicellular region of the paraventricular nucleus,
where CRH-li cells reside. Projections from the noradrenergic region (A1)
of the lateral reticular nucleus terminate in the magnocellular regions of
the paraventricular nucleus and in the supraoptic nucleus in the regions
where AVP-li cells reside. Adrenergic cells in the Cl and Cz regions of the
lateral reticular nucleus and dorsal motor nucleus, respectively, project to
the parvicellular regions of the paraventricular nucleus. There are neural
connections between the parvicellular and magnocellular divisions of the
paraventricular nucleus and between the paraventricular nucleus and the
supraoptic nucleus. AVP is present in large neurons in the supraoptic and
paraventricular nuclei that project to the neural lobe and in small
paraventricular neurons (where it is co-stored with CRH) that project to the
median eminence. The ascending noradrenergic tracts may thus regulate AVP
secretion into median eminence and neural lobe capillaries, for transport
locally to the adenohypophysis and for transport distantly to target organs
(kidneys, systemic arterioles). CRH is present in axon terminals whose cell
bodies lie in the parvicellular divisions of the paraventricular neurons.
It is released by axon terminals lying in the median eminence and is
carried to the pars distalis to stimulate ACTH release.
In the pars
distalis, the increase in AVP and CRH secretion under hypotensive conditions
has a synergistic effect on ACTH release, thus
maximizing ACTH secretion and the stimulation of the adrenal cortex to
secrete cortisol. The AVP released from the neural lobe is carried by
systemic vascular routes to receptors on precapillary arterioles and to
renal parenchymal cells to elevate mean arterial blood pressure and to
increase renal water conservation, respectively. In addition, AVP secreted
by the neural lobe may be carried by capillary routes to the adjacent pars
distalis to stimulate ACTH release. In this manner a coordinated
neuroendocrine response to a single stimulus, hypotension, may be elicited
by the pituitary gland.
Neural Output
from the Periventricular Hypothalamus
AVP-containing
neurons in the paraventricular nucleus project caudally to terminate in the
dorsal motor nucleus of the vagus on noradrenergic cells. A loop is thus
formed, with noradrenergic cells in the dorsal motor nucleus of the vagus
projecting to AVPcontaining neurons in the paraventricular nucleus, and
AVPcontaining neurons projecting back to the dorsal motor nucleus of the
vagus. In addition, paraventricular neurons containing oxytocin project to
brain stem sites and to the intermediolateral cell column of the spinal
cord-the site of the final common pathway for the sympathetic (visceral)
efferent system. Although the details of this system are far from clear, the
paraventricular nucleus would appear to be a site for the integration of
neuroendocrine and autonomic mechanisms.
Levels of
Integration in the
Neuroendocrine System
Sites for
regulating the response of specific functional adenohypophyseal cell
populations and for integrating the responses of several populations of
adenohypophyseal cells are found at several levels but understood well at
only a few. At the level of the adenohypophyseal cell, feedback responsive
to the level of peripheral target hormones occurs. For example, ACTH
secretion is depressed by elevated cortisol levels. TSH secretion is
depressed by elevated levels of thyroid hormone. Other hormones also
regulate adenohypophyseal cell function. For example, epinephrine (from the
adrenal medulla) stimulates ACTH secretion from corticotropes. The role of
cytokines, produced locally or at a distance, in the regulation of
adenohypophyseal function is only now being recognized. Adenohypophyseal
secretions have a paracrine as well as an endocrine function and influence
the function of neighboring adenohypophyseal cells as well as the secretion
of distant targets. Adenohypophyseal cells also respond to neurosecretions
from the median eminence and neural lobe, as the entire neurohypophyseal
capillary bed serves as the final common pathway to the adenohypophysis.
Specific releasing and inhibiting hormones regulate the activity of specific
adenohypophyseal cell populations. Within the median eminence, there is a
morphologic integration of separate neurosecretory systems to form
functional regions. Reproduction is mediated laterally in the median
eminence, whereas neurohormones involved in the regulation of the internal
milieu, metabolism, and response to stress are segregated medially.
Paracrine interactions between neighboring terminals are mediated by co-stored peptide hormones and transmitters. Such interactions are probably
important in the modulation of hypophysiotropic hormone release. At the
level of the hypothalamic nuclei, feedback mechanisms operate in the case of
CRF-li and GnRH-li neurons, which respond to the levels of circulating
steroid hormones. In addition, homotropic neurons synapse with themselves
to make ultrashort feedback loops. Synaptic connection between SRIF and GRH
neurons suggests another type of reciprocal relationship. The organization
of input into the hypothalamus is at this time dimly understood, and no
organizing conceptual framework has been constructed.
Ascending
catecholamine systems (with and without co-stored NPY) have been demonstrated
to synapse with hypothalamic magnocellular and parvicellular neurosecretory
neurons that secret AVP and CRH, respectively. This input is now known to be
excitatory.
For the time
being, the surgeon's scope of endeavour is limited to direct approaches to
the pituitary to normalize its function. The understanding that releasing
hormone systems do not require direct contact with their target cells and
that these systems project toward the ventricular system in their transit
from their sites of origin will permit investigation of the possibilities of
transplanting the pituitary into the sella turcica or even into the
ventricular system. Even transplantation of hypothalamic neurosecretory
cell systems may be possible. Further clarification of the organization of
the hypothalamus and of its input may make it possible to use stereotactic
procedures to modify pituitary function. In the future it is to be expected
that the pituitary surgeon will employ a broad range of procedures in the
brain as well as in the pituitary gland to treat disorders of the
neuroendocrine system.
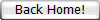 | |